The technology could be particularly useful for situations when
the ball is blocked from view, such as goal-line rushing attempts
when the ball carrier is often buried at the bottom of a pile of
players. The technology could also be useful for tracking the
forward progress of the ball, or for helping viewers follow the ball
during games with low visibility – such as games played during heavy
snow. Video of the technology in action can be seen here.
Previous attempts to design technology that tracks the position of a
football have used high frequency radio waves. But these high
frequency waves are absorbed by players and can be thwarted by the
complex physical environment of a football stadium. Because the
technology would be most useful in pile-ups, when the ball is
obscured by players, these high-frequency approaches aren’t
practical – the absorbed radio waves would result in incorrect or
incomplete data on where the ball is located.
“But low frequency magnetic fields don’t interact very strongly with
the human body, so they are not affected by the players on the field
or the stadium environment,” says Dr. David Ricketts, an associate
professor of electrical and computer engineering at NC State and
senior author of a paper describing the research. “This is part of
what makes our new approach effective.
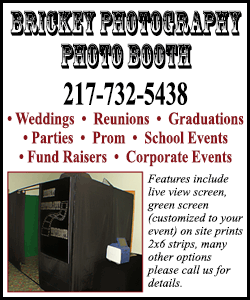 The researchers designed and built a low frequency transmitter that
is integrated into a football, and is within the standard deviation
of accepted professional football weights. In other words, the
football that has the built-in transmitter could be used in a
National Football League game. Antennas, placed around the football
field, receive signals from the transmitter and track its location.
But the researchers also had to address another complicating factor.
When low frequency magnetic fields come into contact with the earth
– such as the playing surface – the ground essentially absorbs the
magnetic field and re-emits it. This secondary field interacts with
the original field and confuses the antennas, which can throw off
the tracking system’s accuracy.
“We realized that we could use a technique developed in the 1960s
called complex image theory,” says Dr. Darmindra Arumugam, lead
author of the paper and a former Ph.D. student at Carnegie Mellon
now at NASA’s Jet Propulsion Laboratory. “Complex image theory
allows us to account for the secondary fields generated by the earth
and compensate for them in our model.”
[to top of second column] |
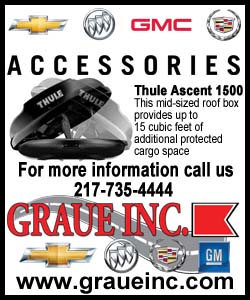
“We’re still fine-tuning the system, but our goal is to get the
precision down to half the length of a football, which is the
estimated margin of error for establishing the placement of the
football using eyesight alone,” Ricketts says.
The paper, “Three-Dimensional Position and Orientation Measurements
using Magnetoquasistatic Fields and Complex Image Theory,” was
published online in June in IEEE Antennas and Propagation Magazine.
Co-authors include Dr. Dan Stancil, head of NC State’s Department of
Electrical and Computer Engineering; and Dr. Joshua Griffin,
formerly of Disney Research. The research stems, in part, from
previous work done by the researchers while at Carnegie Mellon, with
funding from Disney Research.
[Text received; MATT SHIPMAN, NEWS
SERVICES, NCSU]
“Three-Dimensional Position and
Orientation Measurements using Magnetoquasistatic Fields and Complex
Image Theory”
Authors: Darmindra D. Arumugam, Carnegie Mellon University
and NASA Jet Propulsion Laboratory; Joshua D. Griffin, Disney
Research; Daniel D. Stancil and David S. Ricketts, North Carolina
State University
Published: June 2014, IEEE Antennas and Propagation Magazine
Abstract: Coupling between an emitting electrically-small
circular current-carrying loop and seven receiving loops, all placed
outside a grid, located above the earth and within the
magnetoquasistatic region, was measured for three-dimensional (3D)
positions and orientations of the emitting loop over an area of up
to 27.43 m x 27.43 m. Inverting the theoretical expression for the
voltage measured at the terminals of the receiving loops to
determine 3D position and orientation resulted in a mean and median
geometric position error of 0.77 m and 0.71 m, respectively, an
inclination orientation mean and median error of 9.67° and 8.24°,
respectively, and an azimuthal orientation mean and median error of
2.84° and 2.25°, respectively.
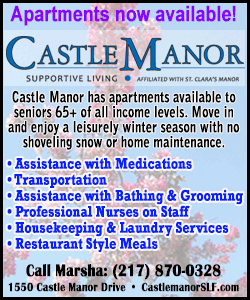 |